Various industries are trying to solve time and processing power consuming problems using quantum computers to unlock valuable applications of quantum computing. The phenomena of quantum entanglement comes useful to cut down on the time and computing power to process information transfer between qubits. Entanglement enables tasks such as quantum cryptography, superdense coding, and teleportation.
Read our article on quantum computers to learn more about what quantum computers are and how they work. For more on quantum entanglement:
What is quantum entanglement?
Quantum entanglement is the state where two systems are so strongly correlated that gaining information about one system will give immediate information about the other no matter how far apart these systems are.
This phenomena baffled scientists like Einstein who called it “a spooky action at a distant” because it violates the rule saying that no information can be transmitted faster than the speed of light. However, further research validated entanglement using photons and electrons.
How is entanglement used in quantum computing?
In quantum computers, changing the state of an entangled qubit will change the state of the paired qubit immediately. Therefore, entanglement improves the processing speed of quantum computers. Doubling the number of qubits will not necessarily double the number of processes since processing one qubit will reveal information about multiple qubits (i.e. the entangled qubits).
According to research, quantum entanglement is necessary for a quantum algorithm to offer an exponential speed-up over classical computations.
Applications of entanglement in quantum computing
Simple 2-qubit entanglement pairs (EPR) have a few identified applications in quantum computing, including:
Superdense coding
In simple words, superdense coding is the process of transporting 2 classical bits of information using 1 entangled qubit. Superdense coding can:
- Allow user to send ahead of time half of what will be needed to reconstruct a classical message ahead of time, which let’s the user transmit at double speed until the pre-delivered qubits run out.
- Convert high-latency bandwidth into low-latency bandwidth by sending half of the information over the high latency channel to support the information coming over the low latency channel.
- Double classical capacity in one direction of a two-way quantum channel (e.g. converting a 2-way quantum channel with bandwidth B (in both directions) into a one-way classical channel with bandwidth 2B).
Quantum cryptography
Cryptography is the process of exchanging information between two parties using an encrypted code and a deciphering key to decrypt the message.
The key to cryptography is to provide a secure channel between 2 parties. Entanglement enables that. If two systems are purely entangled that means they are correlated with each other (i.e. when one changes, the other also changes) and no third party shares this correlation.
Additionally, quantum cryptography benefits from the no-cloning theorem which states that: “it is impossible to create an independent and identical copy of an arbitrary unknown quantum state”. Therefore, it is theoretically impossible to copy data encoded in a quantum state.
For more feel free to read our research on quantum cryptography.
Quantum teleportation
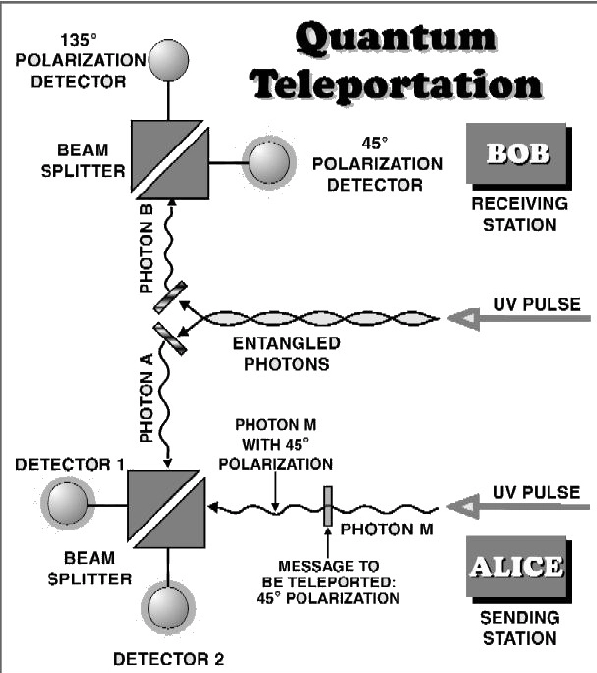
Quantum teleportation is also the process of exchanging quantum information such as photons, atoms, electrons, and superconducting circuits between two parties. Research suggests that teleportation allows QCs to work in parallel and use less electricity reducing the power consumption up to 100 to 1000 times.
The difference between quantum teleportation and quantum cryptography is:
- quantum teleportation exchanges “quantum” information over a classical channel
- quantum cryptography exchanges “classical” information over a quantum channel
Challenges that currently face quantum teleportation are:
- the volume of teleported information
- the amount of quantum information shared between the sender and receiver has before teleportation.
- The sender should have one of the qubits of the pair and the receiver the other qubit of the pair
- The strength of prior correlation between the sender and the receiver qubits increases the capacity of a quantum channel
- teleportation circuit noise acting on the quantum channels
For more on quantum computing
To learn where quantum computing is applicable, feel free to read Top 20+ Quantum Computing Applications / Use Cases
You might also find these articles useful:
If you are interested in learning more about the QC ecosystem, feel free to check our data-driven list of quantum computing companies.
Comments
Your email address will not be published. All fields are required.